Measuring Players’ Emotions Through Biometrics: The Power of Psychophysiology in Games User Research
- 18 marca, 2024
- Joanna Dolata
As a game developer or publisher, you probably know that emotions are crucial to player experience. They impact how players perceive games, what motivates them, and how they behave. Emotions also help players remember the game and the experience; they play a vital role in whether players will purchase the game. With 14,531 games released on Steam in 2023, it’s more important than ever to evoke emotions in players. Your ability to do so can make or break your game. So, how can you be sure that your game, teaser, trailer, or gameplay movie will win the crowd?
You may do it the traditional way…
Game User Researchers commonly use observation, surveys, and interviews to evaluate players’ emotions. However, these methods have both advantages and drawbacks. Surveys and interviews help obtain feedback on a player’s experiences, but they rely on the player’s memory, which can result in lost information due to forgetting. Additionally, responses can be biased due to recall bias, where players remember their experiences better towards the end of the gameplay session rather than at the beginning. Administering surveys or interviews during gameplay can minimize this bias but may interfere with the player’s natural behavior. Furthermore, players may respond in a socially desirable way or portray themselves in a better light. Players might also incorrectly identify the cause of an emotion due to misattribution or generalize an emotion to the whole experience rather than to the element that sparked that emotion.
To understand a particular subject, it is best to use information gathered from various sources to strengthen the credibility of research findings. Therefore, it is advantageous to supplement subjective methods, focusing on players’ conscious attitudes, with more objective psychophysiological measures.
… but it might be better to use the psychophysiological way
Psychophysiology is a fascinating field of study that offers unique and valuable insights into the complex workings of the human mind. By measuring physiological signals like changes in skin conductance, muscle activity, brainwaves, and heart rhythm, we can better understand players’ psychological processes in ways that were once impossible.
These signals are spontaneous, involuntary, and difficult to fake, which makes them incredibly reliable and unbiased sources of information.
The researcher psychologist can access it in real-time throughout the gameplay session—it isn’t dependent on the player’s memory. This technology allows us to record these signals in real-time with minimal impact on the player’s natural behavior. It is extremely precise and can pick up responses smaller than what the human eye can detect, so it’s more accurate than observation in particular usage cases. With the power of psychophysiology, we’re unlocking new levels of precision and understanding how players react to games.
However, these measures have limitations. Directly mapping a psychological state to a physiological effect is impossible, as one physiological response may be associated with many psychological states.
For example, a faster heartbeat and clammy hands might be the body’s response to seeing something scary but also to seeing someone attractive. It’s important to remember that no psychophysiological method to date allows researchers to read players’ minds. While physiological measurements can provide helpful insights into players’ psychology, it’s crucial to remember that researchers have to infer psychological states based on physiological signals. This is why the physiological data must be analyzed in context by qualified researchers with knowledge of psychology, followed by in-depth interviews or surveys.
First, know how we measure emotions in modern psychology
Russell’s circumplex model of affect is a crucial framework for studying emotions in psychophysiological research. The model represents emotions using two dimensions: valence and arousal. Valence refers to the positive or negative direction of an emotion, while arousal (also known as excitement) indicates the intensity of an emotion, whether it is high or low. This model helps us understand emotions due to the interaction between these two dimensions. For example, emotions like anger and sadness have an unpleasant valence, but while sadness is a low-arousal emotion, anger is a high-arousal emotion.
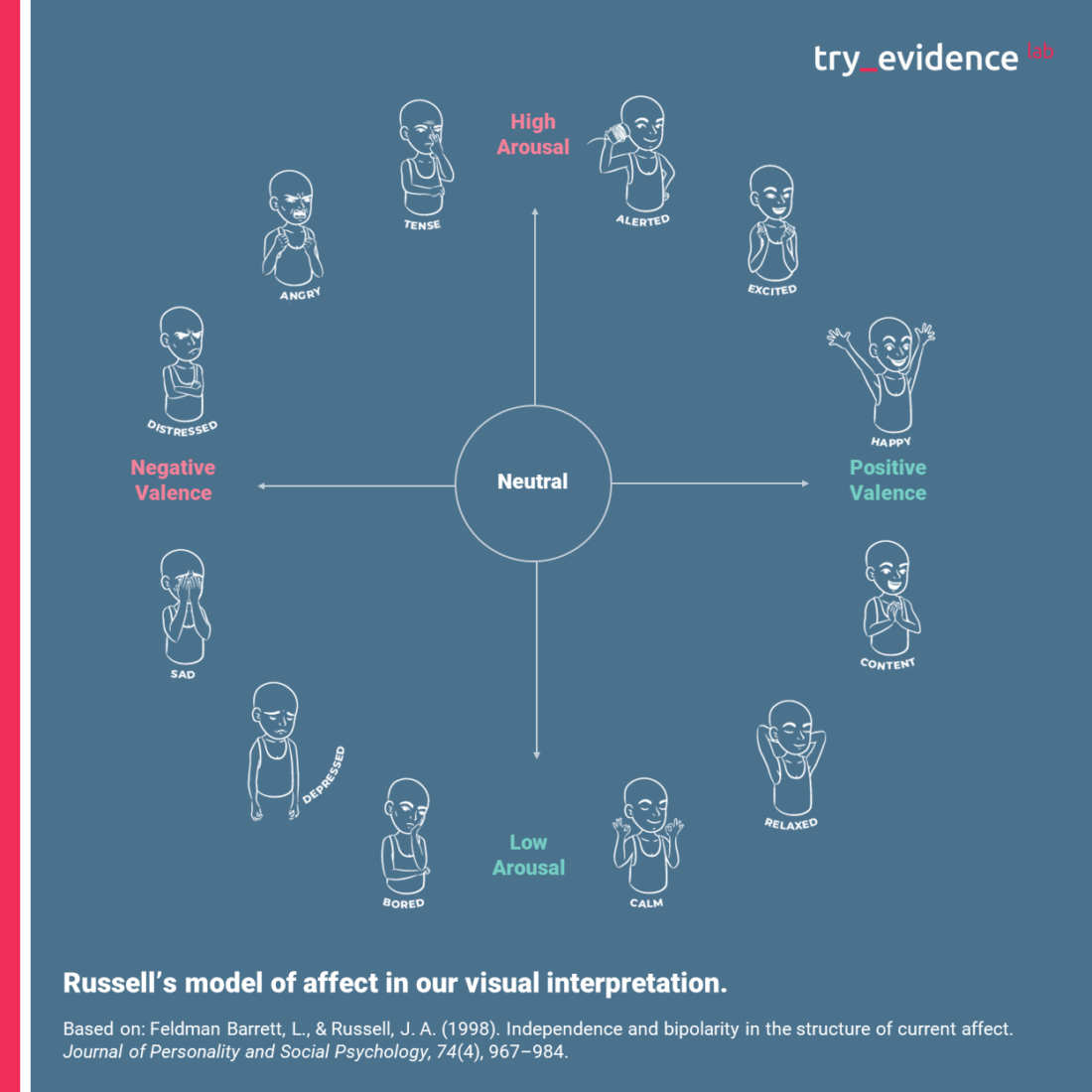
The popularity of Russell’s model in psychophysiological research is due to the limitations of physiological responses in measuring emotions. While psychophysiological instruments cannot identify specific emotions, they can measure signals associated with either valence or arousal. From this data, it is possible to make inferences about the emotions felt by the individual.
With this in mind, we can now examine how this model interprets signals from our bodies.
Second, understand how emotions translate to physiology
Due to the scope of this article, I will briefly present only the most popular types of psychophysiological measurements. At the end of this article, I will provide a list of sources for those seeking more information on these methods.
Electromyography EMG
As previously mentioned, most physiological signals correspond to only one of the two dimensions – valence or arousal. The most prevalent method for measuring the former is electromyography (EMG).
Put simply, EMG measures whether or not a muscle is active. More specifically, it measures the electrical activation of muscle tissue and its contraction. The most common method of studying valence in Games User Research is by measuring facial EMG, which is the activation of specific muscles associated with emotional facial expressions.
For example, the brow muscle is associated with negative valence because it’s activated when we frown.
Conversely, the lower eyelid and cheek muscles are used to index positive valence because they’re involved in smiling.
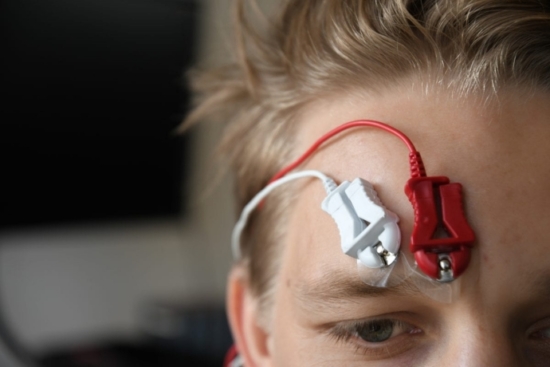
EMG’s definite advantage is that it picks up even slight facial expressions that wouldn’t be observable to the naked eye. Unfortunately, this sensitivity might be a double-edged sword. The electrodes measure the activity of muscles of interest to the researchers and any nearby muscle activity.
Consequently, players can’t talk, laugh, or chew during the measurement to avoid interfering with the data, which could make it unusable. This can make using facial EMG very uncomfortable during long gameplay periods.
Additionally, measuring facial EMG requires the attachment of electrodes to the player’s face, which might be intrusive and uncomfortable for some participants. Moreover, facial EMG may be difficult to measure in a non-clinical setting because it requires precision and care with electrode placement.
Respiration
Psychophysiology provides various methods for measuring emotional arousal, such as studying respiration, cardiovascular activity, and electrodermal activity. I’d like to briefly summarize these measures, starting with respiration.
Respiration can be a source of various data types, obtained by measuring, amongst others, respiratory rate, respiratory variability, depth of breath, or even sigh rates. Usually, a higher respiration rate indicates high arousal, while a decreased respiration rate is associated with low arousal. However, high arousal can also manifest in respiratory variability, such as irregular rhythm of breath, quick variations, and temporary pauses in breathing.
Researchers and GUR practitioners have successfully captured breathing activity with a stretch sensor around the player’s chest. However, stretch sensors are sensitive to movement, such as shifting position or talking, which is a drawback, especially during longer gameplay sessions.
Heart rate (HR)
Another way to gauge emotional arousal involves monitoring the activity of the cardiovascular system, which encompasses the heart, blood vessels, and blood. Many cardiovascular measures are of interest from a psychophysiological standpoint, such as heart rate (HR), interbeat interval (IBI), heart rate variability (HRV), and blood volume pulse (BVP). However, I will focus only on heart rate in this article because it’s more frequently used in psychophysiological research.
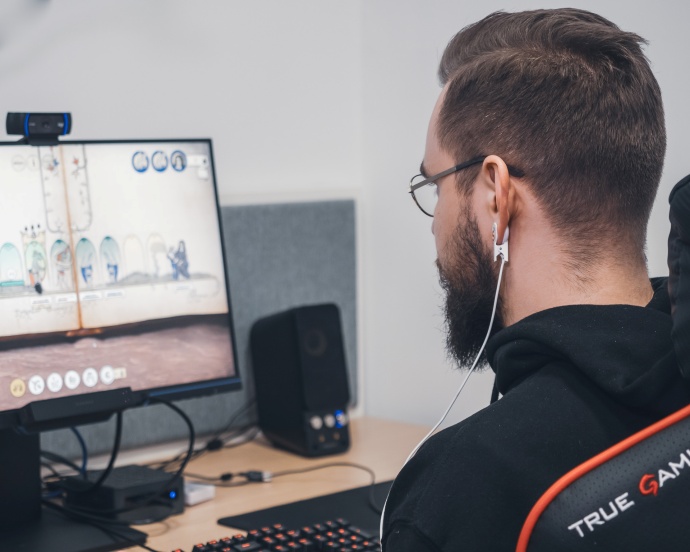
Heart rate (HR) refers to the number of the heart’s contractions per minute. In other words, it’s the measure of the heartbeat’s frequency. Increased HR can be an indicator of increased emotional arousal. Additionally, research has found that HR increases occur during stressful events or experiences of shock, fear, and anger. What’s more, HR can also be used as an indicator of valence, especially in combination with skin temperature measurements.
We must remember that interpreting the cardiovascular system’s signals in the context of the game can be challenging due to various bodily processes regulating its functioning.
Pupillometry
Pupillometry measures changes in pupil diameter (dilation and constriction). These changes are linked to visual and non-visual stimuli, which means that the pupil can change its diameter in reaction to changes in light intensity, but also to mental or emotional processes. Pupil dilation can indicate that the player finds a stimulus emotionally arousing, regardless of its positive or negative valence.
Pupillometry has the advantage of being a non-invasive measurement that can be quickly taken alongside eye movement. However, it is essential to note that game graphics changes, such as a variation in light conditions or color, can also cause fluctuations in pupil diameter.
Electrodermal Activity
The last measure of emotional arousal that I want to introduce, but certainly not the least, is electrodermal activity (EDA). EDA refers to the skin’s electrical conductivity resulting from differences in sweat production in specific sweat glands. Unlike other sweat glands, whose primary function is to moderate body temperature, these respond principally to psychological stimulation. They are primarily located in the palms of the hands and soles of the feet but are also present on the shoulders, wrists, forehead, and neck. Sometimes, it’s called Galvanic Skin Response (GSR).
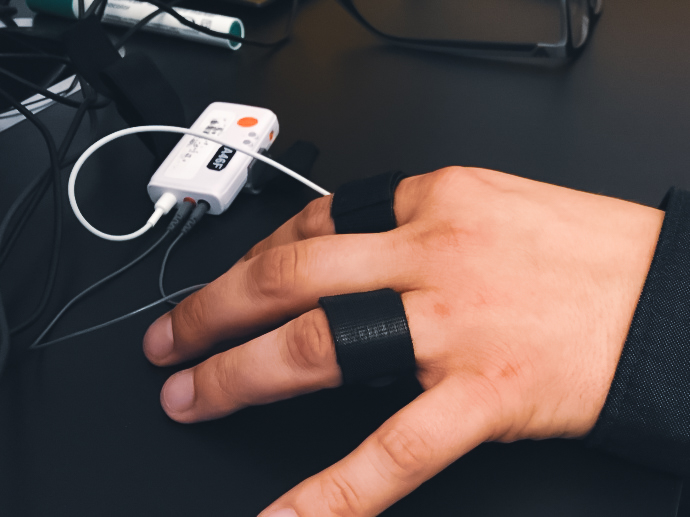
EDA is perhaps one of the most established measures of emotional arousal and is widely recognized in psychophysiology as having an almost one-to-one relationship with arousal. While EDA is praised for its reliability and accuracy, when analyzing the response to a direct event, we have to account for delayed reaction time in response to a stimulus and for a period of recovery time afterward, during which no further event responses will be detected.
Moreover, since EDA is primarily measured by the electrodes attached to hands, players’ movement while manipulating a game controller might compromise the signal. Fortunately, researchers have successfully experimented with alternative electrode placement sites or electrode attachment methods.
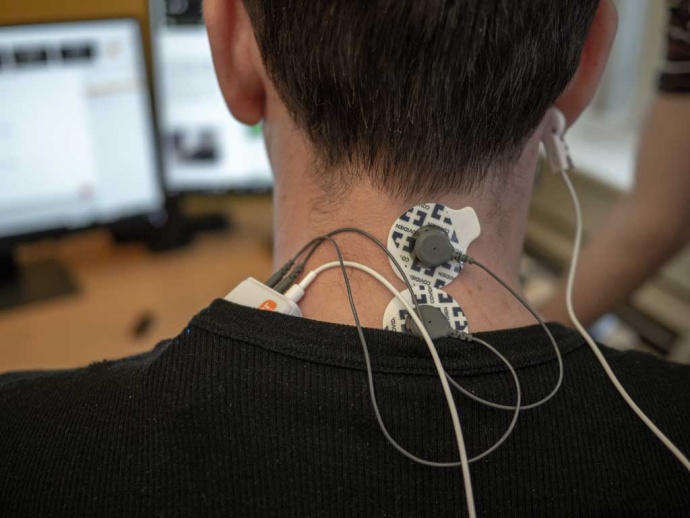
Electroencephalography (EEG)
EEG measures signals generated by neural activity in the brain using electrodes placed on the scalp. Contrary to many myths, EEG doesn’t work like sci-fi technology that would let researchers into peoples’ minds and allow them to read their thoughts.
Then how does it work? Simply put, brain activity is processed using two basic parameters: amplitude (how large the signal is) and frequency (how fast the signal cycles). This allows us to detect patterns in brainwave activity, such as delta, theta, alpha, beta, and gamma activity. These patterns, also known as bands, are related to distinct cognitive, affective, or attentional processes.
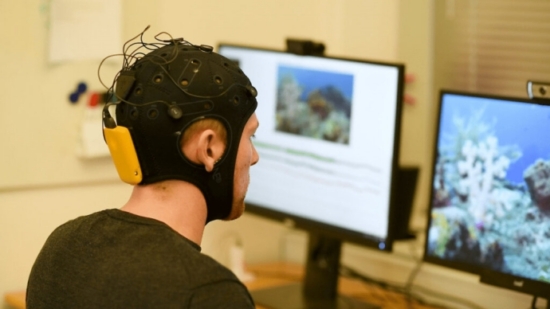
While EEG isn’t a method to measure emotions in the sense of Russell’s model, it nonetheless can give valuable insights into players’ attitudes. We can gain insight into the approach or withdrawal-related tendencies in behavior and emotions by measuring the frontal asymmetry index. Research has found that an increased activity of the left frontal brain is linked to a tendency to approach a stimulus. In contrast, higher engagement of the right frontal brain indicates a tendency to withdraw from a stimulus.
Like other methods, EEG has drawbacks that must be considered before research. Generally, researchers use an EEG cap or headset consisting of wet electrodes to make electrode placement easier and mitigate motion artifacts. You can probably imagine wet hair’s effect on players’ comfort level. Furthermore, EEG measurements are prone to artifacts caused by head movement, making them unsuitable for studying active games or long gameplay periods.
Eye tracking
Finally, I’d like to briefly mention one more psychophysiological method, though not directly related to measuring emotions, which can be successfully combined with other measures to obtain even more valuable insights. As we have covered it in our other article, I will keep it short and sweet.
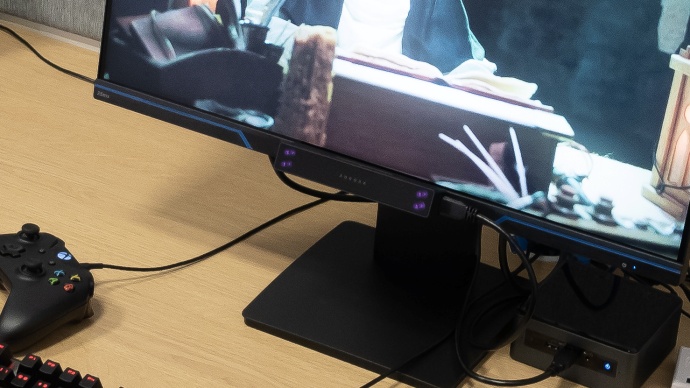
Eye tracking is a method that allows us to track eye movement and gaze to inform us where and for how long the player looked and how the player’s eyes were moving on the screen. While eye tracking can provide insight into a player’s visual attention, solely tracking gaze positions only reveals a little about the cognitive processes and the emotional states that influence eye movements. That’s why, to get the most from eye tracking, combining it with additional data sources, for example, with previously mentioned psychophysiological methods can be beneficial.
Psychophysiology in practice – real-life application
In our lab, we have successfully used various psychophysiological measures to investigate players’ experiences even further. For example, in one of our studies, we compared two trailers for upcoming games, Hellblade: Senua’s Sacrifice and Godfall, to determine which one was more emotionally arousing and attractive to players.
By combining EDA (GSR) with eye tracking, we were able to identify emotional arousal patterns and scenes that triggered emotional activation and track the actual focus of players’ gazes.
In addition, we expanded our understanding by surveying an additional means of gathering information. The survey provided us with insights into how appealing the trailer was to the audience and their willingness to play the game after watching it.
Similarly, Pierre Chalfoun and Jonathan Dankoff have described the study they carried out at Ubisoft’s user research lab on the Far Cry 4 demo trailer. Their work focused on identifying the most emotionally engaging moments in the trailer, assessing its attractiveness, and evaluating the understanding of introduced features. By combining EDA with self-report measures, they found that the co-op feature needed to be adequately introduced and showcased.
They used their findings to refine the trailer through an iterative process, using feedback from each study to modify it before the next test.
Another interesting example of applying psychophysiological methods in assessing trailers comes from studying horror movie trailers. The team at iMotions conducted a small study to determine which of the three trailers was the scariest. They combined eye tracking, EDA, EEG (frontal asymmetry), and self-reports to assess visual attention, emotional arousal, approach vs. avoidance motivation, and each trailer’s likeability and scariness, respectively.
They found that the scariest trailer, as rated by the viewers, was also the most emotionally arousing. Moreover, it elicited the highest behavioral approach motivation,
which may seem counterintuitive as we expect people to be more likely to avoid fear-inducing situations.
However, it’s essential to keep in mind that EEG can’t tell us what’s causing this motivation. It could be triggered by the trailer itself, the context in which it’s being watched, the viewer’s thoughts and feelings towards the trailer, or a combination of all these factors. Nevertheless, by analyzing these findings in the context of the purpose of a movie trailer, the researchers hypothesized that the approach motivation occurred because viewers felt interested in seeing this movie in the cinema. Their hypothesis was confirmed by the additional data obtained from viewers’ self-reports.
However, evaluating trailers is one of many ways to utilize psychophysiological methods in game research. In another one of our studies,
we focused on video game characters and more precisely, on their attractiveness to the players. By measuring EDA, we determined which heroes and heroines caused a high level of arousal in the players.
Not surprisingly, The Witcher’s Geralt of Rivia was the most stimulating male character. We could hypothesize on potential sources of emotional arousal based on which elements drew players’ attention as captured by eye tracking. This research methodology could be effectively applied to character design evaluation.
Psychophysiological methods can also be used on a larger scale, as demonstrated in Macromill’s study of Capcom’s Resident Evil 3 (2020).
Their team used heart rate and EDA as indicators of excitement and vigilance to understand better how the players received the game’s core concepts (survival x horror).
Researchers divided the game into 12 chapters and analyzed the average HR and EDA for each one. This way, they could determine which chapters evoked intense fear and panic in players and which didn’t deliver the intended experience. They learned that one chapter, where a group of zombies chased a character, was less scary for players than anticipated. Additionally, researchers found that fear prompted increased vigilance in players. These findings pushed Capcom to reassess how people feel fear and why they confront it, consequently returning to the crucial question of what horror is.
Takeaways
Emotions are crucial to player experience and impact player behavior and game sales. Leading voices in the Game User Research area agree that combining traditional methods with studying human psychophysiology offers a gateway toward a better understanding of players’ behavior and experience. By accessing human emotions at a more basic, unconscious level, we can gain more accurate insights, which better inform design decisions.
The increased accessibility of physiological sensors has expanded the use of psychophysiological research beyond academic settings into commercial settings. Sensors have become more affordable and more accessible to use outside of clinical settings. Commercially available research software, such as iMotions, which we use in our laboratory, has also made many aspects of psychophysiological analysis faster and more convenient. However, fully automated AI-powered emotion detection models have yet to be made available.
Currently, we use our knowledge of human psychology, its connections to physiology, and its limitations to infer players’ emotions based on human body signals.
This approach reduces susceptibility to bias and is less dependent on the player’s memory, self-esteem, and honesty.
Psychophysiological measures such as changes in skin conductance, muscle activity, brainwaves, and heart rhythm are reliable and unbiased sources of information.
They can be accessed in real time with minimal impact on the player’s natural behavior. The most established ways to measure emotions in Game User Research are electrodermal activity and, to a lesser extent, cardiac activity.
*
There is no surefire way to guarantee that your game, teaser, trailer, or gameplay movie will resonate with the crowd. However, as demonstrated by case studies, psychophysiological methods have been effectively employed in Games User Research labs, such as Try Evidence’s facility, to provide a competitive advantage to design and marketing teams. These insights have enabled developers to prioritize and improve aspects crucial to player experience while ensuring that they deliver emotionally engaging and memorable experiences, without compromising on their original goals.
Further reading
If you’d like to read more about the general overview of psychophysiological methods in Game User Research, you’ll find dedicated chapters in “Games User Research” (Drachen, Mirza-Babaei and Nacke), “Game Usability: Advice from the Experts for Advancing UX Strategy and Practice in Videogames” (Isbister and Hodent), “Game Analytics: Maximizing the Value of Player Data” (El-Nast, Drachen and Canossa) and “Emotion in Games. Theory and Praxis” (Karpouzis and Yannakakis).
Other valuable sources of information are research papers such as “A review of the use of psychophysiological methods in game research” (Kivikangas et al., 2011) or „Let’s Get Physiological, Physiological!”: A Systematic Review of Affective Gaming” (Robinson et al., 2020).
For a deeper understanding of the relationship between emotions in psychology and physiology, I’d recommend reading “Autonomic nervous system activity in emotion: a review” by Sylvia Kreibig.
If you want to see how psychophysiological measures are used in an academic setting, these two articles provide a good example: “Correlation between Heart Rate, Electrodermal Activity and Player Experience in First-Person Shooter Games” (Drachen et al., 2010) and “A fuzzy physiological approach for continuously modeling emotion during interaction with play technologies” (Mandryk and Atkins, 2007).
If you want to know which method best suits your game and goals, don’t hesitate to contact us.